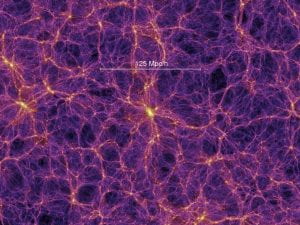
Visualisation of the large scale structure of the universe — from the Millennium simulation. The white line is a distance of approximately 400 million light years; each point of light is a galaxy.
Standard cosmological scenarios propose that the Big Bang was followed immediately by a period of accelerated expansion, or inflation. Inflaton has two roles in shaping the Universe. First and foremost, the rapid growth stretches everything in the early Universe and thus wipes away leftover relics of the Big Bang itself, rendering the primordial Universe almost perfectly smooth and our Universe is indeed smooth and featureless on large scales. However, if the early Universe was perfectly smooth there would have been no “seeds” for the local gravitational growth that eventually lead to galaxy formation. But inflation does not leave the Universe entirely featureless since quantum fluctuations during inflation inject small ripples into the distribution of matter in the early Universe. Between these two effects inflation does an excellent job of providing the starting conditions from which our present Universe evolves.
Literally hundreds of possible inflationary mechanisms have been proposed so a key job for cosmology is to test these alternative hypotheses. One perfectly smooth universe is indistinguishable from another perfectly smooth universe so the observable differences between inflationary models lie in the patterns of primordial ripples they produce. We can infer these from studies of galaxy clustering and the properties of the hot and cold spots we see in the microwave background. From these measurements it seems that each time the Universe grows by a factor of 10 the typical size of the resulting ripples drops by around 4% – an observable parameterised by a quantity called “spectral index”.
The same mechanism that generates ripples in the material that fills the primordial Universe also generates fluctuations in the fabric of space itself, which would manifest themselves in the present-day Universe as a steady background of gravitational waves. The amplitude of these gravitational waves is often expressed relative to the ripples in the matter fields, and is expressed in terms of a parameter known as the tensor-scalar ratio. Relatively simple models (in the sense of the algebraic complexity of their descriptions) typically produce a gravitational wave background with a ratio of between 10 and 20%. However, recent observations cap this quantity at around 4%, ruling out many once-viable inflationary mechanisms.
Most constraints on inflationary models focus on their predictions for the spectral index and the tensor-scalar ratio and in the coming decade several ambitious experiments will be sensitive to tensor-scalar ratios as small as 0.1%. However, from a theoretical perspective there is no compelling reason to expect that the inflationary mechanism is particularly simple and for many plausible scenarios the gravitational wave background is so small it is be undetectable by any conceivable observational techniques. Consequently, it is very possible that only one of these numbers has a value that will never be measurably different from zero.
We have been focusing on how measurements of the running can illuminate the inflationary phase. With David Parkinson and Benedict Bahr-Kalus, Easther showed that some simple inflationary models with very low tensor-scalar ratio also tend to have a larger running, which increases the chance of detecting one signal if the other is below the threshold of future observatories. Looking at experiments now under construction, Bahr-Kalus, Parkinson and Easther showed there is a good chance that measurements of running will be at least ten times more accurate by 2030, closing in on the range where most inflationary models predict that this parameter will lie.
Inflation is driven by physical processes at energies far beyond the reach of terrestrial experiments such as the Large Hadron Collider. Consequently, these cosmological measurements do not just tell us how the Universe began but also offer insight into fundamental physics at ultrahigh energies so the stakes here could not be higher. Our suspicion is that the running is potentially a key cosmological variable – it is small, but unlike the tensor-scalar ratio it is unlikely to be so small as to be forever undetectable and we are thinking about how to maximise the effectiveness of future cosmological experiments to make the most of this opportunity.
Releated Papers
- Constraining cosmic inflation with observations: Prospects for 2030, Benedict Bahr-Kalus, David Parkinson and Richard Easther, Mon.Not.Roy.Astron.Soc. 520 (2023) 2, 2405-2416 • e-Print: 2212.04115 [astro-ph.CO]
- Running primordial perturbations: Inflationary dynamics and observational constraints, Richard Easther, Benedict Bahr-Kalus and David Parkinson, Phys.Rev.D 106 (2022) 6, L061301 • e-Print: 2112.10922 [astro-ph.CO]
- Inflation and the Scale Dependent Spectral Index: Prospects and Strategies, Peter Adshead, Richard Easther, Jonathan Pritchard and Abraham Loeb JCAP 02 (2011) 021 • e-Print: 1007.3748 [astro-ph.CO]
- Implications of a Running Spectral Index for Slow Roll Inflation, Richard Easther and Hiranya Peiris, JCAP 09 (2006) 010 • e-Print: astro-ph/0604214 [astro-ph]